Welcome!
- 01 Apr 2024
- 1 Minute to read
- DarkLight
- PDF
Welcome!
- Updated on 01 Apr 2024
- 1 Minute to read
- DarkLight
- PDF
Article Summary
Share feedback
Thanks for sharing your feedback!
Welcome to the Radix Technical Documentation
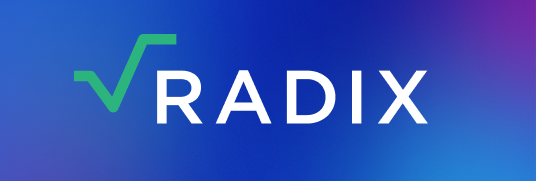
Welcome! Regardless of why you’re here, we recommend that you start your journey by taking 5 minutes to read the essentials about Radix.
If you’re looking for help with a specific topic, please try the search bar above.
If you’re not sure where to go next, try the link that best describes your situation:
Was this article helpful?